Increasing the accessibility of life-changing treatments
Tags
Cell and gene therapies (CGTs) offer hope for patients suffering from a range of cancers and rare diseases. This relatively new class of treatments has the potential to address the underlying causes of disease, compared to traditional approaches which often address only symptoms. However, they are not readily affordable or consequently available to many patients.
There is a need to increase the accessibility of these therapies and there are multiple factors that will drive more widescale uptake. The manufacture of therapies and scaling approaches will reduce the cost burden associated with a manual process requiring highly trained individuals. Putting patients at the centre of this journey will not only keep them better informed of their treatment plans, but will also reduce the number of setbacks seen at the clinical trial stage, making the route to market easier. The development of these treatments can be complemented by new in vitro models that more accurately mimic in vivo environments, allowing more representative data to be collected at the early therapy stages. They might also identify a wider range of health conditions that could benefit from this type of treatment. Finally, understanding the commercial landscape – from regulatory pathways to supply chain logistics, and reimbursement strategies – will facilitate more of these therapies moving from research to widescale use in the clinic.
Scaling the manufacturing process
To provide accessible treatments to a large patient group, the manufacturing process needs to be scaled seamlessly across the value chain from early-stage research, through process development, clinical trials and into commercial production. There is an interesting contradiction within this scaling process that needs to be carefully managed. Towards the earlier stages of development, a large amount of flexibility is required as the appropriate culture protocols are established, whereas these protocols need to be locked down as the therapies transition to manufacture at Contract Manufacturing Organisations (CMOs) under Good Manufacturing Practice (GMP) guidelines. This is the case for both allogenic therapies, where companies are looking to scale-up; and autologous therapies, where scale-out is necessary to produce ‘batch of one’ therapies.
One of the key enablers for scaling manufacture and showing comparability of products is the use of in line quality controls – either through integration of available sensors or by collecting data on alternative parameters analogous to cell growth. There will also be characterisation of starting materials to "tweak" production systems to optimise manufacturing runs. This will not only help define the final product release criteria but will also build a large dataset that can be systematically analysed to better understand the optimal manufacturing conditions. Managing these large datasets is not trivial and is increasingly important as therapies scale. It is important to start collecting as much data as early as possible, especially when working in orphan indications where the path to trials and approvals may be relatively short. It is also vital to collect, store, easily retrieve, and analyse data across different pieces of equipment, at different stages of the development process. In laboratories where multiple therapies will be produced for many patients this can only be achieved through collaboration which will require therapy developers, Contract Development and Manufacturing Organisations (CDMOs) and equipment manufacturers to work more closely.
One of the biggest challenges to the widescale adoption of these therapies is the cost of treatment. Costs associated with a complex manufacturing process, which is labour intensive and run by highly trained individuals, can generally be reduced through automation. There will be different approaches to cost reduction for autologous and allogenic therapies, as companies look to either scale out and increase the number of bioreactors that can be run in parallel to produce “batch of one” therapies or scale up to increase the overall product yield that can be obtained from a single donor. Although likely to be cheaper in the long run, allogeneic approaches may have several layers of costs as developers make drug substance cell banks which require greater testing prior to generating the final drug product.
Increasing Patient centricity
As CGTs begin to be tested in an increasing number of clinical trials, with some successfully reaching the market, the involvement of patients to refine the therapy models and journey becomes key. To date there are over 1,000 CGT clinical trials ongoing or planned to begin in 20211.
Cell and cell-mediated gene therapies require a long time to be administered to patients. This is particularly the case for autologous therapies where the cells sourced from a patient are engineered and/or expanded prior to being injected back into the same individual several days or weeks later. Often this process requires subjects who are unwell to travel to specialised clinical centres creating logistical challenges, exacerbating health issues, and incurring excess costs. In this sense, more sites could be designated for CGT administration to reduce the burden on patients. Especially in autologous therapies such as CAR-T, it is fundamental to identify the correct treatment and administration times over the disease progression. To this end, generating online registries for patients already under the care of healthcare providers could allow for quicker, more efficient recruitment. The end-to-end - or vein-to-vein - process would be faster and cheaper with more efficient communication between the different stakeholders (e.g., sponsor, manufacturing facilities, clinical centres, supply chain etc.) which, in turn, would positively impact the patient experience. The key players can achieve the aforementioned goal by adopting technologies and software that enable the tracking of the drug product across the different stages from apheresis through to storage at the clinical sites. Patients can directly be informed via their personal devices and invited to the clinical centres at the right time.
To further improve the patient journey, patients could be more involved in the decision-making process leading to the clinical trial design. Companies could consider the idea of creating patient advisory boards to actively participate in the development of products and clinical trial design. Furthermore, partnerships with patients’ advocacy groups could also prove helpful for patients to better understand the clinical trial outcomes and raise awareness. This would benefit both recruitment and retention.
Using complementary in vitro models
There is also scope to innovate in new pre-clinical and clinical studies. In recent years, a number of scientific publications reported the generation of three-dimensional in vitro models resembling human organs. Importantly, a study published in 2018 showed how 3D human models of colorectal cancer could recapitulate patient responses to chemotherapy drugs thus laying the foundation for the use of these innovative platforms in personalised medicine2. An organoid is defined as a three-dimensional structure derived from (pluripotent) stem cells, progenitor and/or differentiated cells that self-organise through cell-cell and cell-matrix interactions to recapitulate aspects of the native tissue architecture and function in vitro3.
Due to limitations in replicating the complexity of certain tissues with 2D cultures and in vivo models, organoids have acquired an exponential importance over the past two decades. Since several biological and logistic hurdles limit the use of primary cells, stem cell-derived organoids have gained a predominant role in the scientific community. Furthermore, biomedical research both in academia and in industry is more compliant to the principle of the 3Rs4. Utilising 3D stem cell-derived human models would reduce the use of animals in pre-clinical studies, if not fully replace them, while also avoiding invasive procedures (e.g., biopsies).
Such platforms find application in developmental studies, drug discovery and novel gene therapy testing. The latter represents one the most important applications as it lays the foundation for the replacement of damaged tissues with genetically corrected stem-cell derived organoids in the context of personalised and precise medicine. Additionally, organoids are widely utilised for disease modelling to investigate the pathophysiology and study phenotypic changes to exogenous cells in a model of administration into host tissues.
New technologies that allow for high speed and large-scale production of organoids are emerging with leading companies spanning from the U.S. through to Europe including STEMCELL Technologies, DefiniGen, Cellesce Ltd, Merck and many others. The faster manufacturing process along with the usefulness of such platforms in reducing time and costs for drug discovery, are projecting the global organoids and spheroids market to reach $2.47 billion US by 2028. The academic and research institutes are expected to hold 68.8% of the largest revenue share in 2021 and projections for the next 5 years pose research centres as the key end users. Intestinal, hepatic and biliary organoids are of particular interest, as indicated by the sales and publications growth recorded for these products over the past few years5.
The rapidly growing organoid market is also generating increased interest and expansion in other markets. One of them regards the production of biocompatible scaffolds and biomaterials, as shown by the growing number of companies focusing on these products (e.g., Jellagen, Prellis Biologics, R&D Systems by Bio-Techne, etc.). Another interesting emerging market is linked to image and data analysis. The generation of multiple types of complex organoids along with the innovation in optical setups and omics techniques is providing researchers with new, more extensive raw data that requires novel analytics tools and platforms.
Enabling market adoption
As CGT becomes more mainstream, companies will need to navigate evolving regulatory pathways, understand complex supply chains, and develop new commercial models. The map of each stakeholder in the therapy process is complex, linking the patient, physicians, manufacturing sites, logistics companies and payers in ways not seen with other therapies.
Regulations are evolving to consider how current GMPs and Chemistry Manufacturing and Controls (CMCs) can be applied to these complex therapies. Product characterisation and CMCs can be more challenging to define compared to traditional small molecule drugs, as inconsistencies in the input materials due to patient age, disease progression and underlying health conditions can lead to variability in the output which needs to be accounted for. To fully understand the regulatory pathways, companies should work closely with regulatory bodies early in the product development cycle.
Increasing collaboration between stakeholders across the value chain will be key to getting therapies on the market. Production runs to manufacture materials at CDMOs to support clinical trials or commercial use can take months to plan. This requires sponsors to have a thorough understanding of the clinical trial pathway, patient recruitment strategy and regulatory approval timing to ensure the maximum value can be extracted from the trials. The sponsor of the clinical study will also need to commit a significant amount of time to manage the supply chain, ensure the correct reagents are available when required, and that full batches of reagents are available to reduce variability in the therapy.
Additionally, the high cost of these treatments raises the question of reimbursement levels. This is calculated by incremental cost effectivess ratio (ICER) analysis, looking at both the Quality Adjusted Life Years (QALY) and the current cost of treatment. There are well defined limits on the amounts that will be reimbursed, which vary by geographies and by insurance companies in the US. Companies should be considering these guidelines from the early product development stages to understand if these therapies are likely to be commercially viable. Therefore, while innovative technologies for a more cost-effective and efficient manufacturing process should be adopted, early contacts with payers, pricing landscape for similar products and new reimbursement strategies (e.g., annuity-based payment, outcomes-based payment or rebate) should be encouraged6.
Summary
The CGT space is rapidly evolving and so is the ecosystem within which it sits. Taken together, these will determine the accessibility of CGTs for patients in various forms ranging from the affordability of the therapies through to the physical delivery of the drug product to the clinical sites. We believe scaling manufacturing, improving market access, patient centricity and leveraging innovative technologies for the pre-clinical and clinical settings are the four key areas to improving accessibility to CGTs.
The technical, regulatory and social innovations outlined in this article are some of the key drivers for a future with more reliable, accessible and affordable CGTs for patients.
This article was first published in European Biopharmaceutical Review
References
1 Visit: Informa Cell and Gene Therapies – Custom Analytics Dashboard brought to you by Pharma Intelligence (citeline.com).
2 Vlachogiannis G et al. Patient-derived organoids model treatment response of metastatic gastrointestinal cancers. Science, 2018 Feb 23;359(6378):920-926.
3 Marsee A, Roos FJM et al. Building consensus on definition and nomenclature of hepatic, pancreatic, and biliary organoids. Cell Stem Cell, 2021 May 6;28(5):816-832.
4 Russell WMS & Burch RL. The principles of humane experimental technique. Wheathampstead (UK): Universities Federation for Animal Welfare, 1959.
5 2021 Analysis and Review: Organoids Market by End User – Biopharmaceutical Companies, Contract Research Organization and Academics and Research Institutes for 2021 – 2031. Future Markets Insights, 2021 Jul 4.
6 Burnell S, Siciliano P, Blunt S. Cell and Gene Therapy in 2040, Mar 2021.
More on cell and gene therapy
Explore more
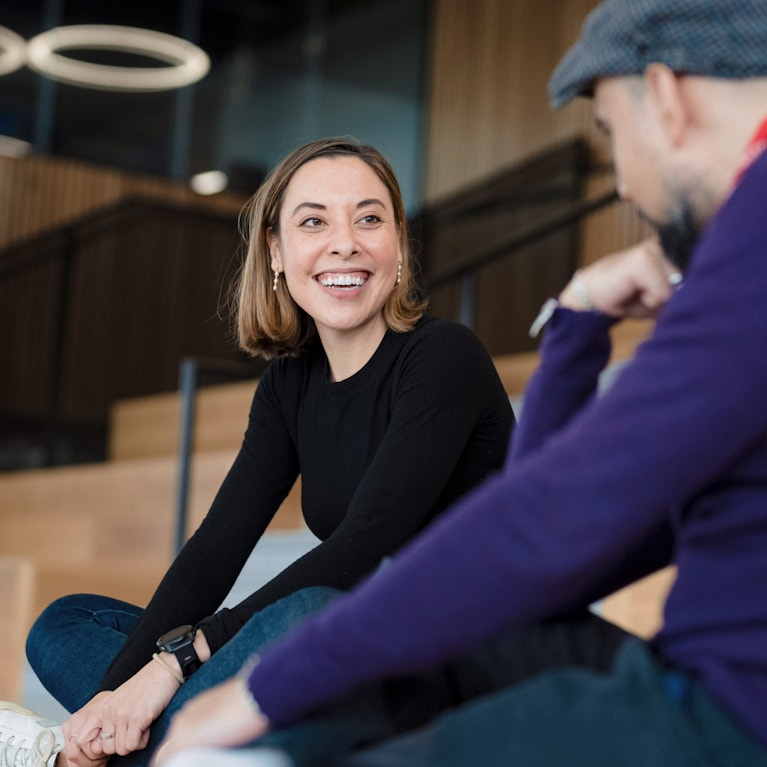